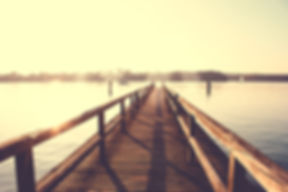
Becca Hedges
Geo 3010-01/Geo 4070-01
Dr. MacLean/Dr. Kaiser
6 Mar. 2017
Summit 2
​
The history of humans on the geologic timescale will show our curiosity of the Earth and what it provides. While we have only been here for a short amount of time, we have accomplished a lot. The more we discover the more our knowledge and technology has advanced. With continued advancements society demands more and more of the Earth. A major commodity of society today is for power. Consumers need power for such things like electricity for their homes using it to charge electronics and use kitchen appliances. Industries need it to run machines in order to create more products which people want. In order to provide power there is a requirement of a fuel source. To provide this we are highly dependent on fossil fuels. These come from deposits of coal, petroleum and natural gas. In recent years we have incorporated renewable energy like solar and wind. Another source we have is from nuclear power. Regardless of which type is used they are all derived from mining for something. Fossil fuels are mined and then burned. Other materials are mined and then used in the building of solar panels and turbines. Uranium is mined for the use of its nuclear fission capabilities. What we are needing to accomplish is clean energy. As it is now fossil fuels are not the answer, they are a huge contributor of greenhouse gas emissions. Renewables are clean energy. However they are dependent on sunny and windy days. Nuclear power is the fuel of the future.
​
Cosmic Creation of Uranium
​
To understand why nuclear power should be continued and expanded we must first explore how it is such a viable option and where it comes from. The most important component is of course uranium. Uranium is a member of the actinide group on the periodic table with an atomic number of 92 and denoted with the symbol U. It is a heavy element with 92 protons and 92 electrons. As with most elements it has its beginnings from the process of stars turning into supernovas. This process is due to the combination of a stars elemental composition, mass, and internal pressure and temperature. When a star reaches the end of its life a chain of reactions will cause an explosion via the process of nuclear fission. Through a process called rapid neutron capture or the r-process, free neutrons created by the supernova can alter into different elements if captured by a different element that can accept it. This must occur in a very short amount of time hence it being termed as rapid. This is how we explain the creation of elements with an atomic number greater than iron which is 26 (Faure, 1998). All of the Uranium in the universe began to exist by this process.
​
As the Earth began to form it was circling in the suns orbit along with cosmic dust and gas. This dust and gas were remains from the Big Bang and other supernova events (Wood, 40). Uranium was contained within the dust and gas. The Earth gained mass by colliding with other objects in its path and began to differentiate into a super-heated heavy metal core. Uranium was one of these heavy elements (Roberts, 2013). According to the World Nuclear Association the abundances of U-235 and U-238 at the time the Earth was formed can be calculated. If all the uranium available in the solar system originated from a single supernova, this event is estimated to have occurred about 6.5 billion years ago. This estimate is obviously older then the formation of the Earth which formed about 4.5 billion years ago (Wood, 41). In reality all uranium in the solar system was likely derived from many supernova events.
​
Uranium Deposits
​
The World Nuclear Association says that uranium is found all over the world and in a variety of geologic settings. The beginnings of the Earth held the uranium within the mantle but today it is more enriched in the crust. This mostly occurs by geologic processes involved with magmatism. Three main igneous events allow for uranium among other elements to be transferred from the mantle to the crust. The first is by the formation of oceanic crust at places like mid-ocean ridges. This is because of decompression melting allowing the magma to rise creating new crust. The second form is from the rise of mantle plumes through the lithosphere which can reach the surface. The third involves subduction zones which creates island and continental arcs (Wood. 42).
​
After the earth experiences these three processes other subsequent geologic process gives us 15 different categories in which uranium deposits are found. They are distinguished by their geologic setting and/or the type of host rock they are located in. These can be igneous, sedimentary, or basin type deposits (World Nuclear Association). This wide diversity of worldwide uranium deposits are due to redox reactions in its 4+ ionic state. In oxidizing conditions uranium is very soluble and reactive. It is most commonly associated with oxygen, hydroxyle, carbonates, sulfates, chlorine, fluorine, phosphorus, and bromine. It also dissolves in acids and reacts with water. When uranium precipitates it is typically caused by a reduction condition. This is due to a change in the O2 levels. Uranium is found to be about 50% of minerals such as sphene, orthite, monazite, zircon, apatite, ilmenite and others (Zachara, 25). It is the most abundant in uranium oxides named uraninite and pitchblende (Mercadier, 264).
​
While we can find uranium deposits in a variety of ways, about 75% of the world’s uranium deposits are derived from 5 of the 15 categories: Intrusive, Polymetallic hematite breccia complex (IOCG), Metasomatite, Proterozoic unconformity, and Sandstone. These deposits came from four major times in our geologic history: In the Hadean (4.55-3.1 Ga) there was an anoxic atmosphere and deposits of uranium were not being created. However, the process of transporting magmas and aqueous fluids enriched with uranium were in progress. During the Archean (3.1-2.2 Ga) the atmosphere began having an increase of free oxygen. There were very limited amounts of uranium being deposited at this time. With the Proterozoic (2.2-0.45Ga) we see the most vital period in which uranium deposits exist. Many different Unconformity, Metasomatite, and IOCG deposits were laid. There was a high amount of free oxygen available in the atmosphere as well as the hydrosphere. Organic rich sediments increased while the first redox reaction deposits began. Throughout the Paleozoic (0.45 Ga-Present) most of the sandstones received hydrothermal-volcanic uranium deposits (Fayak, 3-15). Erosion during any of these periods would also transport uranium in turn creating new deposits.
​
Radioactivity, and Nuclear Fission
​
Uranium was discovered in 1789 by a German scientist named Martin Heinrich Klaproth. He named the element after the planet Uranus (rsc.org). This element is the reason radioactivity was discovered in the first place. In 1897 French physicist Henri Becquerel was researching how light influenced salts. Some salts with uranium were put on a plate and it fogged up. From that it was determined some sort of emissions were being created (Pappas, 2015). Since that time Uranium has become a major player in the role of nuclear energy because of the large energy potential from chemical processes it holds.
​
In 1932 the neutron was discovered. In 1934 Enrico Fermi started bombarding uranium with neutrons. This produced what he thought were elements that could be heavier than uranium. What he didn’t know is that he was actually splitting the atoms into lighter ones. This discovery came in 1938 by physicists Lise Meitner and Otto Frisch. They made sense of the discovery comparing it to a water droplet that becomes elongated eventually splitting into two separate water droplets. They named this new process "fission" after the term "binary fission" used by biologists to describe the division of cells. After this work was published and accepted scientists recognized that the fission reaction also emitted secondary neutrons and a chain reaction could occur. This type of reaction would produce high amounts of energy and a race to create atomic weapons began. Meitner wanted no part in creating weapons, however she did help in the construction of Sweden’s first nuclear reactor (Tretkoff 2007).
​
Because Uranium is naturally radioactive its nucleus is unstable, so the element is in a constant state of decay. It wants a more stable arrangement and has 16 different isotopes, 3 of which occur naturally. Uranium-238 has a half-life of 4.5 billion years, Uranium-235 has a half-life a little over 700 million years and, Uranium-234 has a half-life of 245,500 years. Uranium-234 only occurs from the decay of U-238 (Pappas, 2015). Uranium-238 decays with an alpha emission into thorium-234, which will then decay with a beta emission to protactinium-234, which decays by beta emission to uranium-234, and so on. These daughter products will continue the alpha and beta emission process until it reaches a stable configuration of lead-206 (ieer.org).
​
Nuclear Power
​
In 1942 Enrico Fermi conducted the first controlled nuclear reaction. The US had its first nuclear bomb test in 1945. Now, this is what most people think of when they hear the word nuclear. Bombs and explosions. What comes as a secondary thought is of a power source. During the 1950’s-60’s the number of nuclear power plants were increasing significantly. Uranium is the central element of the nuclear-fuel cycle, with U-235 being the fissile isotope (Zachara, 1.1). This process releases fission products of kinetic energy, gamma radiation and free neutrons. The free neutrons produced can cause additional fission reactions. All of the energy produced from these reactions create heat. This will make steam that powers the turbines to generate electricity. The fuel rods in nuclear reactors are made of tubes with small pellets of uranium dioxide (UO2). Reactors are cooled with water as this process produces such high levels of heat. Reactors also have capabilities to control the rate of fusion or to stop the process of fission all together (Poullikkas, 744). Nuclear power has a very low operating cost. An average cost in the US to generate electricity is about $2.40 per kilowatt-hour. Each nuclear reactor holds the potential of generating power from forty to sixty years. The power created from the nuclear fission is about ten times more powerful than that from fossil fuels. Nuclear is also a very reliable and steady source of energy. In the US as a whole about 20% of our power comes from nuclear power plants (Half-Death).
​
Environmental Hazards and the Yucca Mountain Repository
​
Because of the way uranium is there are radioactive levels located around deposits naturally. This poses health risks to any living thing that will be in contact with that environment. There are many known hazards associated with nuclear power. One of the largest problems with nuclear energy is the environmental impact of mining and milling for the uranium itself. This is not a clean process. Effects from mining and especially the milling process could contaminate soil, water, and air. Uranium mine operations must abide by their safety, testing and reclamation plans to minimize these effects. Once the fuel is used in the reactors, you can’t simply take it to a landfill, it’s radioactive and dangerous. The actual transporting of nuclear fuel and waste to and from plants or storage facilities represents a pollution hazard. Nuclear power plants can create 20 metric tons of nuclear fuel per year, and approximately 2,000 metric tons a year of nuclear waste (Half-Death). This waste holds the potential to consume any compartment that holds it. As discussed above through decay processes uranium will eventually turn into lead, however this takes a countless number of years. Nuclear accidents of any kind can pose serious health effects on the lives of people as well as the environment. The Chernobyl accident that occurred on 26 April 1986 at the Chernobyl Nuclear Power Plant in Ukraine was the worst nuclear accident in history. Its harmful effects on humans and ecology can still be seen today (Osuna).
​
In order for us to be safe and efficient with the use of nuclear power we need a safe place for the storage of the waste produced. The site at Yucca Mountain has been in development since the early 1980’s and so far has had over 8 billion dollars spent on it. This site was chosen because of its geochemistry, rocks characteristics, tectonics, meteorology, costs and socioeconomic impacts. It is one of the most studied and appropriate locations within the US (Nuclear Energy Institute). This site is located in a sparsely populated area and will use about 1100 acres (energy.gov). The storage compartments of the spent rods will be placed into a cask which is double lined with stainless steel. On the ends of the container there are three layers composed of alloy 22. These containers would then be placed on an emplacement pallet and surrounded by a drip shield. For even more extra protection this entire unit is then closed within a perforated stainless steel sheet (EIS Statement).
​
In the world today a terroristic attack is possible. The locations of any nuclear reactor or spent fuel rods is public knowledge. This makes them an easy target. A lot of damage could be caused from such events (www.conserve-energy-future.com). The US has over 100 nuclear plants in operation with more than 1.6 million people living within 75 miles of one (Osuna). Most of them are concentrated in the east. A problem with this is each one of them currently house their own waste because they have nowhere else to put it. If an attack were to happen, local areas would be destroyed. Even more so because storing the waste on site adds more fuel to the fire. This also creates issues even without the risk of terrorism. These sites were never meant to house this waste. They are being held in temporary structures. Some are already seeing the effects. As the radioactive material begins to eat through the containers the local environment is at risk of contamination. Of these sites many of them are located near water sources which as we know, could spread the contamination further.
​
If there is any type of melt down or explosion at these facilities it would contaminate anything in the local area and be limited at the sites of nuclear power plants. In order for there to be a meltdown there must be an active fission process. This would not happen at a repository (Hewitt 133). For an explosion to occur there would need to be something which triggered the event. This is not likely to happen because the repository site has been built to withstand an airplane crashing into the building and other sabotage type events (energy.gov).
​
Even though there has been volcanic eruptions in the past and this location was formed from large explosive volcanic eruptions about 12 million years ago, there is only about a 5% chance of another one occurring within the next 1.8 ×105 years (Ho, 65). Recent studies also show that there is a trend of intrusive activity moving away from the selected site. A benefit that could be created from an intrusion or surface flow is they could create a natural encasement of the repository site (Crowe, 250). This site is positioned 1000 feet above the local groundwater and 1000 ft. below the surface. This area is a dry climate producing only 7.5 inches of rain fall annually (pollutionissues.com). The potential impact is only with an accident occurring in which the radioactive material could seep down into the ground water. The EIS statement has planned for this in the construction of the site by having multiple layers of the outer walls. Being that there are some fault systems in the area the EIS has stated that the building codes will be in compliance of earthquake standards. This is also one way that the perforated stainless steel sheets will be useful. They will serve as an extra barrier if any cave in type events were to happen. The radioactive longevity estimates of all the waste stored here is between 10,000 to one million years. If there were to be a containment leak at the repository during this time, it is estimated it could release 700 millirem per year. For perspective there are residents in Colorado who live with natural radioactive levels in the soil at 700 millirem per year (colorado.gov).
​
Two of the most important aspects of the controversy with Yucca Mountain are the transportation of the waste and of the waste itself. According to The Office of Civilian Radioactive Waste Management National Transportation Plan, worldwide there has been 70,000 metric tons of waste that has been transported, of that 3000 metric tons has been within the US. Thus far there has not been a single disaster. There will be 3 major ways of transporting the waste along designated routes of Railroad, Truck, and Barge. The majority is expected to be by rail. Every shipment will have trained personal and satellite tracking of all shipments. The personal will be expected to inspect all cargo at undisclosed intervals. All containers will be required to pass a drop from a minimum of 30 ft. onto an unyielding surface. They must also withstand being engulfed in a fire producing 1475 degrees F for a minimum of 30 minutes, and must be able to stand being fully submerged in a minimum of 3 ft. of water. (yuccamountain.org).
​
The Yucca Mountain site has a 70,000 metric ton capacity. One concern with this is currently the US has 62,000 tons of waste. At our consumption rate this site will fill up soon. One way to control this aspect is recycling what waste we can. Currently the US has no procedures to re-enrich spent rods or any other type of waste usage. While the US does not do this, places in Europe are making many advances regarding what to do with nuclear waste. In France about 75% of their power comes from nuclear, of that 17% comes from recycled nuclear waste. They reprocess or re-enrich uranium and plutonium fuel rods to recover more fuel that is still available in the nuclear waste products (World Nuclear Association). At the University of Bristol, scientists have found a way to compress and encapsulate radioactive nuclear waste into a diamond like material called Black Diamonds. This process produces a clean battery energy which they say will last for 5000 years or more (Technology and Innovation).
Conclusion
​
The costs that are most associated with nuclear power is in the mining for the uranium and building the reactors. This is a classic case of the benefits outweighing the cost. This power source also gives us the ability to be proactive rather than reactive. While the process of extracting uranium from the earth is not a clean process, production from nuclear reactors are, they do not emit greenhouse gasses, only steam. Using our resources of fossil fuels hits us twice: first, in the mining process and second, with the burning of them. In “Fail-Safe Nuclear Power” by Richard Martin, he states “The world need nuclear energy more than ever if we are to limit climate change if we are to stay within 2 degrees Celsius of warming”. We need a safe place to store the waste produced until such a time comes that it decays to stability or we can recycle the waste into other power options. More research can and should be done on the recycling of nuclear waste. There is more potential there than people realize. The Yucca Mountain repository is designed not only for safe storage but the easy retrieval of the waste containers. If we keep expecting society to continue while making advances, how can we expect to rely on the energy of the past? By reducing even some of the waste that is produced some of the negative impacts of using nuclear power will also be reduced. Having one location in which to study and store waste limits the areas where contamination can occur. It makes more sense to store the nuclear waste in one environment which is designed and constructed for long term storage rather than over 100 different locations in temporary conditions that are not designed for long term storage.
Works Cited
World Nuclear Association http://www.world-nuclear.org/information-library/nuclear-fuel-cycle/uranium-resources/the-cosmic-origins-of-uranium.aspx Web. 27 Feb. 2017
Wood, Bernard. “The Formation and Differentiation of Earth.” Physics Today 64.12 (2011): 40-45. GeoRef. Web. 3 Mar. 2017.
rsc.org. Royal Society of Chemistry 2016. Uranium. http://www.rsc.org/periodic-table/element/92/uranium Web. 27 Feb. 2017
Pappas, Stephanie. “Facts about Uranium”. LiveScience. http://www.livescience.com/39773-facts-about-uranium.html Web. 27 Feb. 2017
Zachara, J., C. Liu, et. al., “A Site-Wide Perspective on Uranium Geochemistry at the Hanford Site”. Pacific Northwest Laboratory of the US Department of Energy. (2007): https://www.pnl.gov/main/publications/external/technical_reports/PNNL-17031.pdf Web. 3 Mar. 2017.
Tretkoff, Ernie. “Discovery of Nuclear Fission”. APS News. 16.11 (2007). Academic Search Premiere. Web. 1 Mar. 2017.
Fayek, Mostafa. “Geochronology of Unconformity related Uranium Deposits”.
Institute for Energy and Environmental Research. http://ieer.org/resource/factsheets/uranium-its-uses-and-hazards/. Web. 28 Feb. 2017.
Poullikkas, Andres. “An Overview of Future Sustainable Nuclear Power Reactors”. International Journal of Energy and Environment. 4.15 (2013): 743-776. GeoRef. Web. 1 Mar. 2017.
Ho, Chih-Hsiang. “Time Trend Analysis of Basaltic Volcanism for the Yucca Mountain Site”. Science Direct. 46.1 (1991): 61-72. GeoRef. Web. 28 Feb. 2017
http://www.sciencedirect.com/science/article/pii/037702739190076C?via%3Dihub
Crowe, Bruce M. “Volcanic Hazzard Assessment for Disposal of High-Level Radioactive Waste”. Google Scholar. Chapter 16:247-266. Web. 28 Feb. 2017. https://www.nap.edu/read/624/chapter/19#258
pollutionissues.com. http://www.pollutionissues.com/Ve-Z/Yucca-Mountain.html. Web. 28 Feb. 2017.
Hewitt, Geoffrey F, John G Coller. “Introduction to Nuclear Power”. Taylor and Francis. 2nd ed: (2000). Google Books. Print. Web. 1 Mar. 2017.
Energy.gov. “EIS”. https://energy.gov/sites/prod/files/EIS-0250-S1-FEIS-01-2008.pdf. Web. 1 Mar. 2017
Colorado.gov. https://www.colorado.gov/pacific/sites/default/files/HM_tenorm-Comments-V_0.pdf. Web. 3 Mar. 2017
Roberts, Greg. “Star Death and the Origin of Uranium”. Stanford. 21 Mar. 2013. Web. 26 Feb. 2017.
YuccaMountain.org. Web. 28 Feb. 2017
www.conserve-energy-future.com. Web. 28 Feb. 2017
"Half-death." The Economist. The Economist Newspaper, 29 Oct. 2015. Web. 12 Feb. 2017.
Faure, Gunter. Principles and applications of geochemistry: a comprehensive textbook for geology students. Upper Saddle River, NJ: Prentice Hall, 1998. Print.
Technology and Innovation. http://secondnexus.com/technology-and-innovation/diamond-batteries/?utm_content=inf_10_1164_2&tse_id=INF_70f4e2c0f57311e6a03c354c456e1db2 1 Feb. 2017. Web. 1 Mar. 2017.
Osuna, Ric. “Subversive Doubt: The Story of Yucca Mountain Nuclear Repository”. YouTube. 16 Feb. 2016. Web. Feb. & March 2017.
Martin, Richard. “Fail Safe Nuclear Power”. MIT Technology Review. 119.5: 38-44. GeoRef. Web. 28 Feb. 2017.
Mercadier, Julian. Et.al. “Origin of Uranium Deposits Revealed by their Rare Earth Element Signature”.
Mercadier, Julien, et. al. "Origin of uranium deposits revealed by their rare earth element signature." Terra Nova 23.4 (2011): 264-69. Web. 1 Mar. 2017